
Slump test and massive wind turbine foundations
The slump test, developed by Duff A. Abrams in the 1910s, is a straightforward method for assessing the consistency of concrete.
In the early days of concrete technology, controlling the flow of concrete was primarily achieved by adjusting the water content. Adding more water improved workability but came at the cost of reduced strength and durability. The slump test emerged as a practical means to evaluate whether excessive water had been incorporated, balancing the need for workability with structural integrity.
Concrete has since evolved into a more complex material. There're lots of different admixtures that affect workability besides the amount of water. While the slump test may no longer provide insights into concrete's strength and durability, it remains one of the most widely employed tests for assessing fresh concrete workability. Its enduring popularity is rooted in its simplicity and effectiveness.
How to perform a Slump Test
Performing a slump test is a straightforward process. You start by filling a metal cone-shaped mold with fresh concrete, and then you remove the cone to observe how much the concrete slumps or settles.
The slump is measured by the difference between the height of the cone and the height of the slumped concrete.
How to interpret the Slump Test results
The higher the slump, the easier the concrete flows.
At first sight, given that we only measure one parameter, the test interpretation may seem straight forward. However, not all slumps are created equal, even if they share the same numerical value.
It's important to evaluate the shape of the concrete. Ideally, we'll expect the concrete to simply subside, keeping more or less to shape. This is called a True Slump.
In some cases, you might observe an inclined sliding plane, where the top portion falls down and ends up beside the bottom. While this may measure the same as a common slump test in terms of height, it conveys entirely different information. This phenomenon, known as shear failure, often occurs when there is either too little paste content or an excess of sand in your concrete mixture.
If the slump simply crumbles and falls apart, this indicates an insufficient quantity of sand in the concrete mix. Sand plays a vital role in giving concrete cohesion, ensuring that it holds together effectively.
Another issue to watch out for is segregation. When you conduct the slump test, and the rock particles remain in a pile while the mortar separates to one or more sides, you're witnessing segregation. This typically occurs when there is an overabundance of a single aggregate size or a group of aggregates that do not blend well together.
Slump Test in different Standards
The America Concrete Institute ACI 211.1 recommends slump ranges for different types of constructions within the civil engineering and building sectors.
Type of construction | Slump [in] | Slump [mm] |
---|---|---|
Reinforced foundation walls and footings | 1 - 3 | 25 - 75 |
Plain footings, caissons, and substructure walls | 1 - 3 | 25 - 75 |
Beams and reinforced walls | 1 - 4 | 25 - 100 |
Building columns | 1 - 4 | 25 - 100 |
Pavements and slabs | 1 - 3 | 25 - 75 |
Mass concrete | 1 - 2 | 25 - 50 |
The European Standard EN 12350-2 distinguishes between 5 classes of consistency depending on the slump test result.
Class | Slump [mm] | Slump [in] |
---|---|---|
S1 | 10 - 40 | ½ - 1½ |
S2 | 50 - 90 | 2 - 3½ |
S3 | 100 - 150 | 4 - 6 |
S4 | 160 - 210 | 6¼ - 8¼ |
S5 | ≥ 220 | ≥ 8¾ |
The British Standard BS 8500-1 provides some guidance recommending slump classes for different concrete uses.
Use of concrete | Slump class | Slump [mm] | Slump [in] |
---|---|---|---|
Kerb bedding and backing | S1 | 10 - 40 | ½ - 1½ |
Floors and hand placed pavements | S2 | 50 - 90 | 2 - 3½ |
Strip footings Mass concrete foundations Blinding RC slabs, beams, walls, and columns Pumped concrete | S3 | 100 - 150 | 4 - 6 |
Trench fill In-situ piling | S4 | 160 - 210 | 6¼ - 8¼ |
Slump needed for Onshore wind turbine foundations
WTG foundations are massive structures with a complex reinforcement layout consisting of hundreds of reinforcing bars.
The center of the foundation is densely packed with rebars, anchor bolts, an embedment ring, and ducts. At the same time, this region is where the tower and foundation connect, making it crucial for the effective transmission of forces within the system. It's important that the fresh concrete properly surrounds all reinforcement and other elements to ensure a correct stress distribution throughout the foundation's design life.
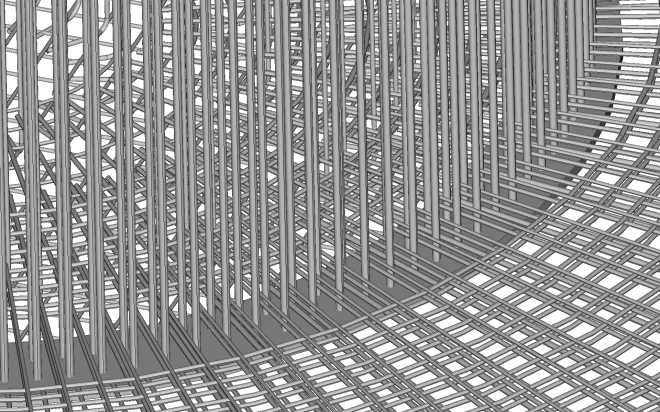
On the other hand, shallow wind turbine foundations always have a tapered slab to optimize the concrete volume. Some times this slope can reach up to 30% (16.7 degrees) or even more. If the fresh concrete flows too much, it may be difficult to correctly execute this slope.
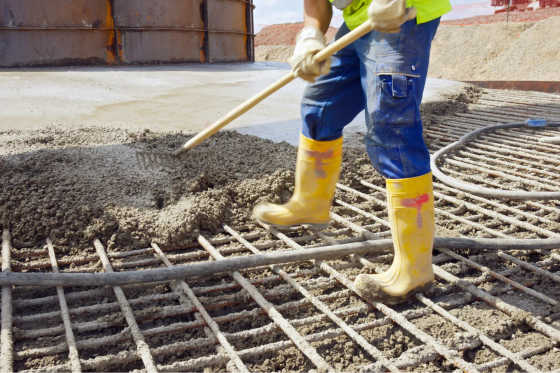
WTG foundations commonly require a slump between 10 and 15 cm (4 to 6 inches), which corresponds to a slump class S3 according to the Eurocodes. This is in line with the recommendation mentioned before in the British Standard.
However, it's important to consider the two potential challenges mentioned earlier:
- The concrete at the center of the slab may need larger slumps to ensure that fresh concrete can properly flow and surround all the rebars and anchor cage elements.
- Slabs with pronounced slopes may need a concrete with smaller slumps so it can maintain the correct shape before hardening.